Subscribe to Genomely for the latest discoveries and in-depth analyses in your inbox.
Thank you to our subscribers for your continued support and passion for science!
The bacterial flagellum is a remarkable propeller-like machine that allows a basic cellular function—movement. The ability of bacteria to move toward food or away from danger is a key attribute of their success. While rotation is known to be bi-directional, the structural and mechanistic basis for rotational switching from one direction to another (typically a response based on chemical cues) has historically evaded researchers. Here, we cover the latest breakthroughs in understanding how bacteria move, including switching rotational directions.
![The Bacterial Flagellum [IMAGE] | EurekAlert! Science News Releases The Bacterial Flagellum [IMAGE] | EurekAlert! Science News Releases](https://substackcdn.com/image/fetch/w_1456,c_limit,f_auto,q_auto:good,fl_progressive:steep/https%3A%2F%2Fsubstack-post-media.s3.amazonaws.com%2Fpublic%2Fimages%2Ffc4304ec-f2a3-480b-badc-286b596ea4d6_1440x1669.jpeg)
The Flagellar Motor: A Century of Discovery
In the 1970s, Howard Berg and colleagues made the groundbreaking discovery that bacterial motility is based on the rotation of the flagellar filament, driven by a rotary motor (the basal body). Despite significant structural variations across species, the core mechanisms of flagellar motility are largely conserved. The flagellar filament's rotation is coupled to a large cytoplasmic ring structure, the C-ring, whose rotation is powered by peripheral protein complexes called stators.
A vital aspect of motility is chemotaxis—the ability to change swimming direction in response to chemical attractants or repellents. This phenomenon hinges on the flagellar motor's ability to switch its rotational direction. Recent advances in cryo-electron microscopy (cryoEM) have allowed researchers to study intact flagellar structures at high resolution. These studies revealed that the stator complexes function as rotary motors, challenging previous models of how the flagellum rotates. The new model posits that the C-ring is a passive cog, actively driven by the smaller stator cogs on its periphery.
Determining the Mechanism of Directional Switching
CryoEM images of the C-ring's structure revealed that the FliG protein's domains, which interact with the stators, rotated 180 degrees. Snapshots of the clockwise and counterclockwise C-rings in their stator-engaged states facilitated this finding. Specifically, the FliG protein's remodeling altered the C-ring teeth, changing the stator interaction surface from the inside to the outside (or vice versa). In the default configuration, the stator interacts with the C-ring's external face, and the small cog's clockwise rotation drives the large cog's counterclockwise rotation. The binding of chemotactic regulators to the C-ring changes its structure so that the stator now interacts with the inner face, causing the same small cog rotation to drive the large cog in the opposite direction.
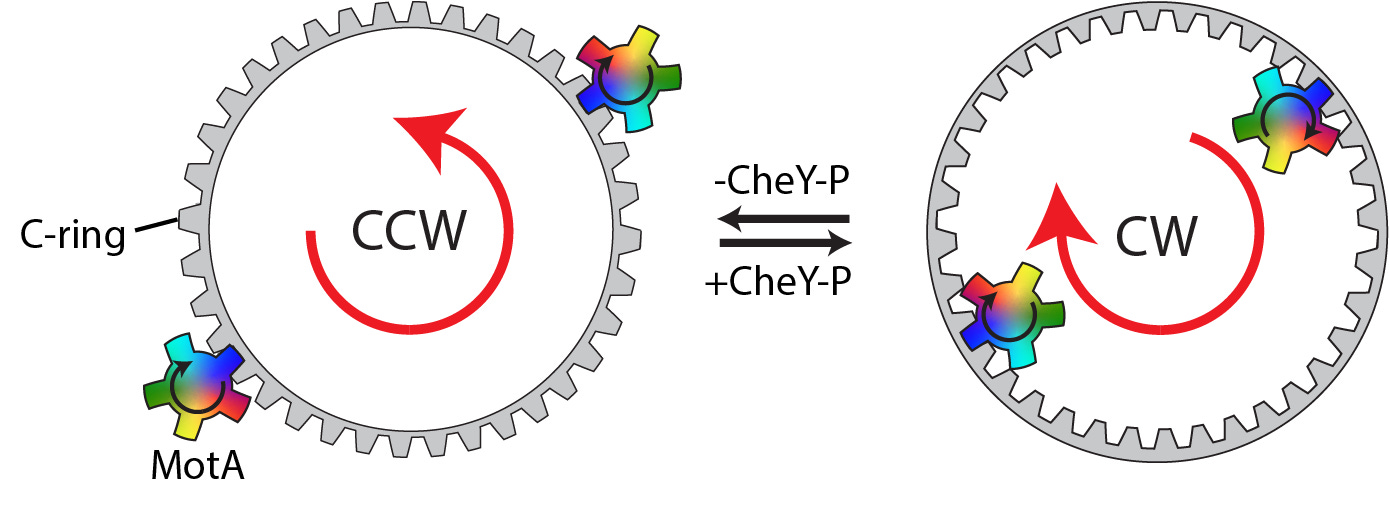
A Model of Sensitivity and Precision
Researchers also found high cooperativity of this switching. Specifically, the stator's rotation primes the C-ring subunits for switching by pushing them toward their switched conformation. Furthermore, the C-ring subunits are intricately intertwined, preventing neighboring subunits from existing in different states. This means regulator-induced changes quickly propagate around the ring from a single binding site. These mechanisms ensure exquisite sensitivity to environmental signals and minimize the chance of opposing forces acting on different sides of the C-ring cog, making rotational switching an "all or nothing" process.
References
Johnson, S., Deme, J.C., Furlong, E.J. et al. Structural basis of directional switching by the bacterial flagellum. Nat Microbiol (2024). https://doi.org/10.1038/s41564-024-01630-z